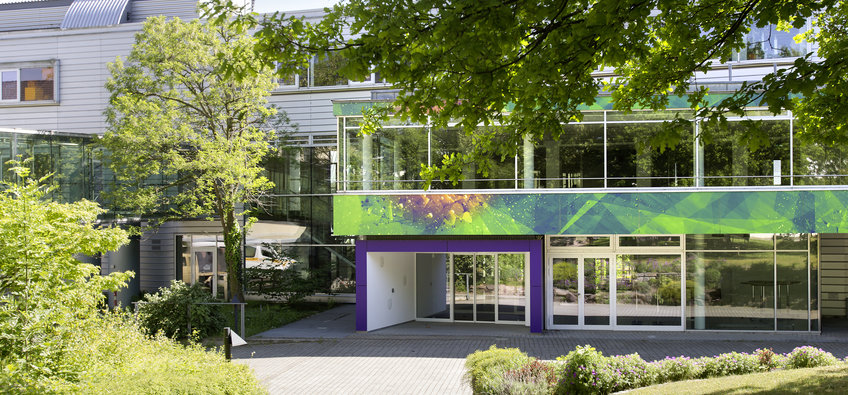
Max Planck Institute of Microstructure Physics
The electronics of the future could operate with light instead of electricity – or a combination of the two. As yet, no ideal light sources are available for this, however, nor are fibre optics fully developed. The development of such materials is one of the challenges that the scientists at the Max Planck Institute for Microstructure Physics in Halle have taken on. They investigate how the microstructure and nanostructure of metallic compounds affect their physical properties, for example how they behave as fibre optics or their magnetic characteristics. Their research concentrates on materials in low dimensions, for instance in a two-dimensional thin layer, a virtually one-dimensional nanowire or a minute heap of atoms, which physicists call a quantum dot and which, in some respects, resembles a single atom.
Contact
Weinberg 206120 Halle (Saale)
Phone: +49 345 5582-50
PhD opportunities
This institute has an International Max Planck Research School (IMPRS):
IMPRS for Science and Technology of Nano-SystemsIn addition, there is the possibility of individual doctoral research. Please contact the directors or research group leaders at the Institute.