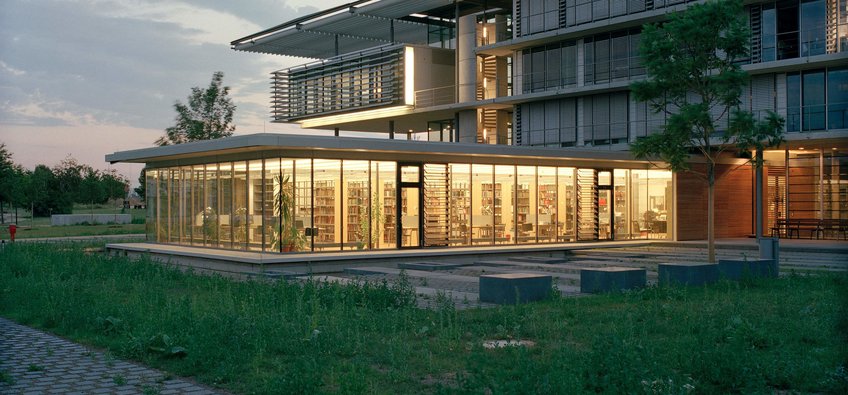
Max Planck Institute of Biophysics
At the Max Planck Institute of Biophysics, research is mainly focused on proteins that are embedded in or associated with biological membranes. Among other things, membrane proteins act as channels, transporters or molecular sensors for the exchange of substances and information between the cell and its environment, but they are also important for transport within cells. The Institute's scientists use electron microscopy and X-ray crystallography to analyse the structure of these proteins. In an ideal complement to the experimental investigations, these molecular processes are also modelled in the computer, in order to describe them quantitatively and gain a detailed understanding of the underlying mechanisms.
Contact
Max-von-Laue-Straße 360438 Frankfurt am Main
Phone: +49 69 6303-0
Fax: +49 69 6303-4502
PhD opportunities
This institute has an International Max Planck Research School (IMPRS):
IMPRS on Cellular BiophysicsIn addition, there is the possibility of individual doctoral research. Please contact the directors or research group leaders at the Institute.