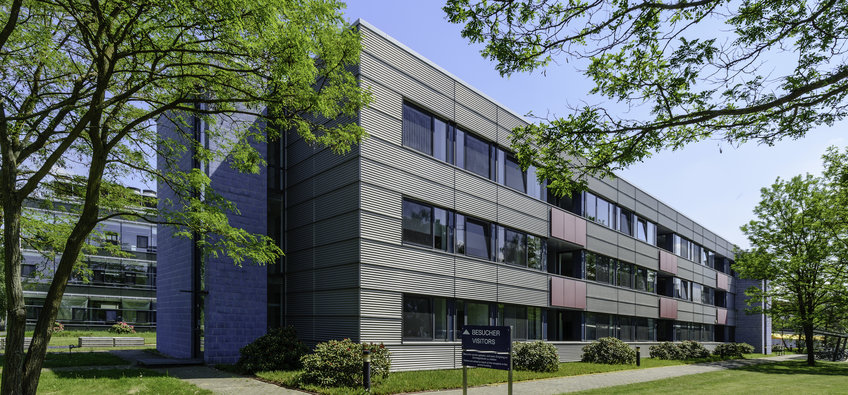
Max Planck Institute for Gravitational Physics
Since its foundation in 1995, the Max Planck Institute for Gravitational Physics (Albert Einstein Institute, AEI) in Potsdam-Golm has established itself as a leading international research center. Its research program covers the entire spectrum of gravitational physics: from the giant dimensions of the Universe to the tiny scales of strings. The AEI is the only institute in the world that brings together all of these key research fields. AEI scientists investigate the mathematical foundations of Einstein's theory of space-time and gravitation. Others work towards the unification of both fundamental theories of physics – general relativity and quantum mechanics – into a theory of quantum gravity. Other scientists do research on gravitational waves, neutron stars, black holes, the two-body problem in general relativity, and the analytical and numerical solutions of Einstein's equations. They are thus contributing to a new era of astronomy, which began on September 14, 2015 with the first direct detection of gravitational waves on Earth by LIGO.
Central research topics of the other AEI branch in Hannover are the development and implementation of data analysis algorithms for a variety of gravitational wave sources as well as work on gravitational wave detectors.
Contact
Am Mühlenberg 114476 Potsdam-Golm
Phone: +49 331 567-70
Fax: +49 331 567-7298
PhD opportunities
This institute has an International Max Planck Research School (IMPRS):
IMPRS on Gravitational Wave AstronomyIn addition, there is the possibility of individual doctoral research. Please contact the directors or research group leaders at the Institute.