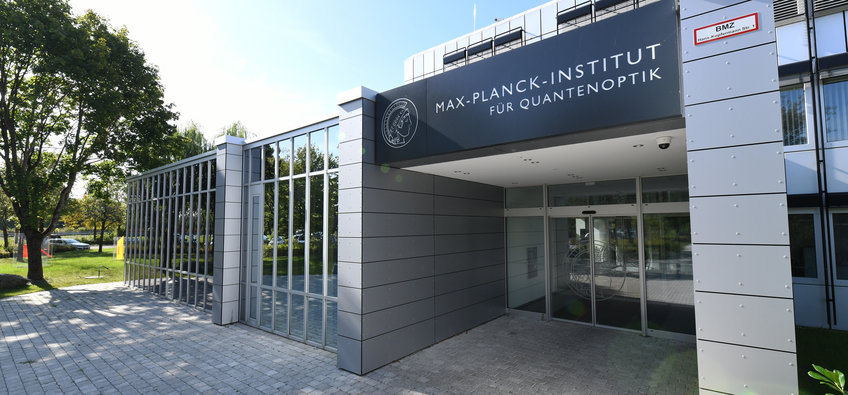
Max Planck Institute of Quantum Optics
The work of the Max Planck Institute of Quantum Optics focuses on investigating the quantum world with laser light. The physicists employ complex facilities comprising many optical components, such as mirrors and lenses, to trap and manipulate systems of quantum particles right down to individual atoms or molecules. They are thus laying the foundations for the quantum computers of the future, at the same time gaining insight into new types and exotic states of quantum matter. By generating ultra-short and high-intensity flashes of light the scientists can observe and control the motion of electrons in atoms. These experiments pave the way for extremely fast electronics and new types of radiation sources for medical diagnostics and therapy.
Contact
Hans-Kopfermann-Str. 185748 Garching
Phone: +49 89 32905-0
Fax: +49 89 32905-200
PhD opportunities
This institute has several International Max Planck Research Schools (IMPRS):
IMPRS on Advanced Photon ScienceIMPRS for Quantum Science and Technology
In addition, there is the possibility of individual doctoral research. Please contact the directors or research group leaders at the Institute.