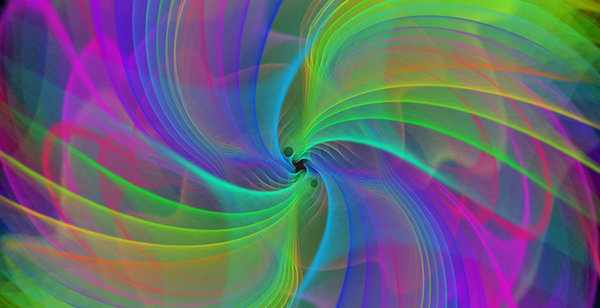
The long road towards evidence
With the discovery of gravitational waves, general relativity has passed another test brilliantly. The official announcement on February 11, 2016 provides a closing chapter for a story that began 100 years ago.
Text: Alexander Blum, Roberto Lalli und Jürgen Renn
The first publication on gravitational waves within the general theory of relativity, entitled “Approximative Integration of the Field Equations of Gravitation,” was written by Albert Einstein and submitted on 22 June 1916 to the Prussian Academy of Sciences in Berlin. Yet for many decades afterwards, Einstein himself, and many others, remained uncertain about the actual, physical existence of these waves.
Collaborative work on the historiography 20th century physics by the Einstein Papers Project at Caltech, the Hebrew University of Jerusalem, and the Max Planck Institute for the History of Science carried out over many years has recently shown that the prediction of gravitational waves emerged as early as February 1916 from an exchange of letters between Albert Einstein and the astronomer Karl Schwarzschild . In these letters Einstein expressed skepticism about their existence. It is remarkable that their significant physical and mathematical work was carried out in the midst of a devastating war, while Schwarzschild served on the Eastern Front.
After Schwarzschild’s death at age 42 in May 1916, most likely as a consequence of a severe auto-immune disease, Einstein returned to the subject of gravitational waves after having received a letter from the astronomer Willem de Sitter. From it Einstein learned that a mathematical obstacle which had prevented him from pursuing Schwarzschild’s line of investigation could be overcome. In June 1916 he published a follow-up paper to his recently formulated theory of the gravitational field in which he predicted the existence of gravitational waves traveling at the speed of light, in analogy with electromagnetic radiation (i.e. light, radio waves, etc.). In it he derived a formula for the emission of gravitational waves. This paper contained a significant error, which Einstein himself corrected in 1918, when he derived a formula for the emission of gravitational waves that, apart from a factor of 2, is still considered to be the correct one. His calculations showed, however, that these waves were too weak to be observed with the technology then available.
Einstein’s calculation involved an approximation that made general relativity look like the well-known theory of the electromagnetic field. This approximation, and the results Einstein derived from it, were criticized by many, most notably by the English astronomer and astrophysicist Arthur Eddington, who otherwise was one of the main proponents of general relativity. Indeed, in 1919 Eddington had spectacularly confirmed another prediction of the theory–the bending of light in the gravitational field of the Sun–making Einstein an international celebrity. Over time, and despite his own calculations, Einstein himself came to doubt the existence of gravitational waves to such an extent that, in 1936, he and his collaborator Nathan Rosen wrote a paper purporting to demonstrate the point. After a referee spotted a mistake in their argument, the paper was finally published in a different journal with a completely different conclusion, leaving open the original question. This fascinating episode is described in great detail in Daniel Kennefick’s book Traveling at the Speed of Thought, which gives an excellent overview of the history of gravitational waves.
Gravitational waves were hardly a hot topic at the time. Indeed, the period from the 1920s until the 1950s has been called the “low water mark of general relativity.” As has been made clear by recent historical investigations jointly undertaken by the Max Planck Institute for the History of Science, the Hebrew University, and the Collected Papers of Albert Einstein, those few physicists working on the subject at the time were more interested in finding a new theory to replace general relativity that might encompass or even explain the new, exciting developments in quantum theory; or in discussing more general issues related to cosmology, such as the evolution of the universe as a whole. And even these few physicists were scattered throughout the world and only rarely exchanged results or ideas. There was, in other words, no recognized field of research called “general relativity (and gravitation)” as there is today, with entire scientific institutions (such as the Max Planck Institute for Gravitational Physics) devoted to it.
This began to change only in the mid-1950s. The “renaissance of general relativity” was made possible by the considerable funding made available to theoretical physics after World War II, as well as by the ability of scientists to cross international boundaries. But the main driving force was the physicists themselves, who recognized the potential for establishing a vibrant community interested in the many aspects of Einstein’s theory. Conferences, journals, and an international society dedicated specifically to general relativity were the result. Among the most pressing of the questions that could now be pursued were the existence and properties of gravitational waves.
Heated discussions about gravitational waves took place at the first international conference entirely dedicated to general relativity in Bern in 1955. Two years later a follow-up conference was held in Chapel Hill, North Carolina. (The report of the conference was first published in 2011 in the Edition Open Sources Series of the Max Planck Institute for the History of Science and the University of Oklahoma). Based on work by the Anglo-Austrian physicist Hermann Bondi and the American Richard Feynman, a broad consensus formed that gravitational waves were real and that they carry energy.
The first attempts to observe the tiny effects produced by such waves were made by Joseph Weber of the University of Maryland. With a few assistants and students, Weber began his experimenting around 1960; after nearly a decade he announced that he had collected convincing evidence. Weber’s work had a significant impact on the scientific community, sparking a series of experiments designed to test his results. . None of these confirmed Weber’s findings, leading to a long and heated controversy that has been analyzed by Harry Collins, who stressed the relevance of social factors on the manner in which scientific controversies over experimental results unfold and end. Although a consensus eventually emerged that no gravitational waves had been observed in Weber’s experiments, new techniques and methodologies were developed that later constituted the basis of larger machines, including LIGO.
In addition to advances in technology, a second prerequisite for the direct detection of gravitational waves in 2016 was a better understanding of their astronomical sources. Indeed, an “indirect” detection of had already been performed in 1974 by closely observing a binary system of two massive stars circling each other which slowly lost energy by emitting gravitational waves, with the result that the stars slow down and move ever closer to each other. The energy loss, observed by Joseph Taylor and Russell Hulse, precisely matched theoretical calculations, earning Hulse and Taylor the 1993 Nobel Prize in physics.
The source of the gravitational waves now detected by LIGO is novel, for it involves the collision of two black holes. The very concept of such a thing as a black hole resulted from a new understanding of Einstein’s equations. Still, the actual existence of black holes only began to be taken seriously after the 1960s, when observations made in the newly established field of radio astronomy (a result of the radio technology developed in the course of radar research in World War II) indicated that such extreme objects really existed in distant galaxies.
Being able to qualitatively explain the properties of such distant, yet incredibly luminous radio sources (quasars) was one of the great successes of general relativity in those years, solidifying its newly-won status as an exciting field of research. Numerical simulations for the behavior of black holes had to be made in order to reach actual predictions. Simulations of this sort required advancing in computing technology as well as theoretical developments in order to follow the evolution of a system over time, as was done most notably by the physicists Richard Arnowitt, Stanley Deser, and Charles Misner. The result was the birth of an entirely new field of research: numerical relativity.
While the discovery of gravitational waves is now rightly heralded as the confirmation of a prediction Einstein made 100 years ago, the likelihood of their existence really only started to gain traction in the 1950s and 1960s, when the great technological, conceptual, and organizational advances of the renaissance of general relativity laid the groundwork for this year’s discovery, and initiated a boom that has lasted to this day and has made possible large-scale, international projects such as LIGO. The groundwork for the establishment of LIGO was prepared close to forty years ago by Rainer Weiss, Ronald Drever, and Kip Thorne. It took more than two decades after the project’s start to obtain significant signals that confirmed ripples in the spacetime structure of the universe and the existence of black holes first speculated on a century ago.
In 2015, the Max Planck Institute of the History of Science initiated a collaborative research project with the Collected Papers of Albert Einstein at Caltech and the Hebrew University in Jerusalem on the multifaceted renaissance period of general relativity and the important role of gravitational waves. So, while gravitational waves, after having been discovered, also promise to provide a new tool with which to observe the universe, the history of this long-controversial subject will be further illuminated as well.