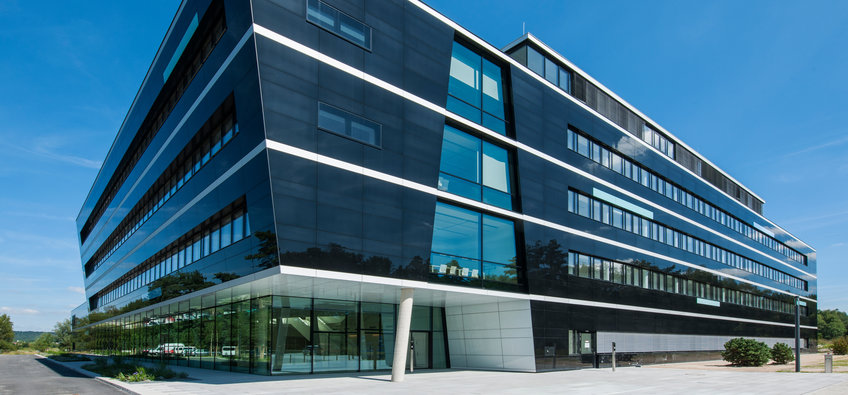
Max Planck Institute for the Science of Light
White light sources being several orders of magnitudes brighter than light bulbs, the manipulation of single photons or the smallest focal point in the world – these are just a few skills mastered or developed by the scientists of the Max Planck Institute for the Science of Light. Their main goal is to control light in all dimensions: in time and space, polarisation – i.e. simply speaking the direction of oscillation – and quantum properties. The knowledge they develop could simplify telecommunication or enable more compact data storage. For this purpose the researchers use novel optical structures like optical glass fibres with a regular lattice of tiny hollow channels along its length. Glass fibres guide light with extremely low losses and can be several kilometres long.
Contact
Staudtstraße 291058 Erlangen
Phone: +49 9131 7133-0
Fax: +49 9131 7133-990
PhD opportunities
This institute has several International Max Planck Research Schools (IMPRS):
IMPRS Physics of LightIMPRS Physics and Medicine
In addition, there is the possibility of individual doctoral research. Please contact the directors or research group leaders at the Institute.